Preface
This groundbreaking publication scrutinizes a comprehensive exploration of climatic conditions in the Philippines. Drawing on meticulous historical observations and cutting-edge climate projections from the sixth phase of the Coupled Model Intercomparison Project (CMIP6), based on a set of Shared Socio-economic Pathways (SSPs), this report offers insights into the future of our nation's climate. Utilizing upgraded, state-of-the-art climate models and new narratives about future societal development, we delve deep into the intricate relationship between climate dynamics and socio-economic factors such as population dynamics, economic trends, land use patterns, and energy consumption. By illuminating the complex interplay of greenhouse gas and aerosol emissions scenarios, we strive to enhance our understanding of how the climate system responds to human influence.
This publication is more than just a compendium of data—it is a crucial resource for environmental planners, policymakers, and concerned individuals dedicated to climate change adaptation and mitigation. It serves as a guiding beacon for national and local government units (LGUs), empowering them to craft robust climate action plans and strategies. In addition to its comprehensive analysis, this publication provides invaluable insights into projected monthly temperature and rainfall trends for municipalities and provinces across the country. Furthermore, it introduces a revised Climate Information Risk Analysis Matrix (CLIRAM) tool, accompanied by a comprehensive guide on its effective utilization.
We are optimistic that this publication will prove instrumental in reducing climate change vulnerability and fostering proactive responses to the challenges ahead. By equipping stakeholders with the knowledge and tools needed to anticipate and address climate change, we can collectively forge a more resilient and sustainable future for all.
CMIP6-BASED Climate Projection in the Philippines Request Form
Preface
This report summarize important findings about the state of the Philippine climate based on the most recent observations, as well as climate projections, which were derived from state-of-the-art climate modles utilizing information from updated future climate scenarios. This report is very timely especially in the formution of local climate change action plans and in the mainstreaming activities of the national and local government units. This may serve as a reference for different usersneeding information in formulating climate change adaptation strategies at the national and local levels.
For interested readers, a comprehensive guide in the proper utilization of the climate projections using PAGASA's newly developed Climate Information Risk Analysis Matrix (CLIRAM) is provided herein. Also included in this report are the summaries of the seasonal climate projection data for useful for thir own applicaitons, and we look forward to everbody's support and cooperation in promoting a climate-resilient country.
PAGASA extends ots deepest gratitude to the following individuals, and the agencies they represent, for their expertise, tireless assistance, and motivation in the generation of the projection data from which this report was based upon: Joseph Daron, Richard Jone, Ian Macadam, Florian Gallo, Ron Kahana, Clair Scannel, David Hein, Simon Tucker, Rosanna Amato, and David Corbeli of the UK met Office Hadley Center; Jack Katzfey, and Dewi Kironoof Commonwealth Scientific and Industrial Research Organisation (CSIRO), Australia; Kevin Hodges of the Department of Meteorology, University of Reading, Reading Unite Kingdom; and Sharon Taylor of the Philippine Rural Reconstruction Movement (PRRM).
Climate Trends and Projection in the Philippines Request Form
Report Summary
The Philippine Atmospheric, Geophysical and Astronomical Services Administration of the Department of Science and Technology (DOST-PAGASA) regularly conducts climate modeling initiatives, in collaboration with domestic and international partners to better understand climate change and its effects in the country. In partnership with the Manila Observatory and the Ateneo de Manila University, DOST-PAGASA took part in the “Analyzing CORDEX-SEA (Coordinated Regional Climate Downscaling Experiment — Southeast Asia) Regional Climate Simulations for Improved Climate Information over the Philippines: SST Influence, Variability and Extremes, Tropical Cyclone Activity” program that aims to generate high-resolution climate change information from multiple state-of-the-art climate models.
In this report, the downscaled historical and projected daily extremes data were used to calculate the projected changes in 24 climate extremes indices for two Representative Concentration Pathways: RCP4.5 and RCP8.5. Historical simulations for the baseline period (1986–2005) served as the threshold. The multi-model ensemble consisted of 12 models consisting of three regional climate models (RCMs) forced with data from 10 global climate models (GCMs) from the Coupled Model Intercomparison Project — Phase 5 (CMIP5) archive. SA-OBS, a daily gridded observational dataset for Southeast Asia based on the Southeast Asian Climate Assessment & Dataset (SACA&D) project was used as the historically observed baseline data.
The Philippine Climate Extremes Report 2020 presents information on historical and projected annual climate extremes indices of the country and demonstrates their relevance to sector-specific climate impacts assessment. This report extends the climate projection information released by DOST-PAGASA in 2018 which used the 10th, 50th and 90th percentile thresholds of temperature and rainfall to describe the average annual and seasonal changes in future climate scenarios. The annual climate extremes indices may be used to identify areas and sectors which are most at risk to climate extremes and thus require rapid disaster risk assessment and climate adaptation planning to minimize current and future impacts. Local government units may use this report in formulating local climate change action plans and mainstreaming of national climate change initiatives.
Since the choice of climate change adaptation strategies is rooted in the proper understanding of the impacts of extreme events, this report also presents a framework for analyzing possible impacts and assessing adaptation options for highly sensitive sectors.
Provincial tables of observed and projected changes in climate extremes are provided in the Annex as reference.
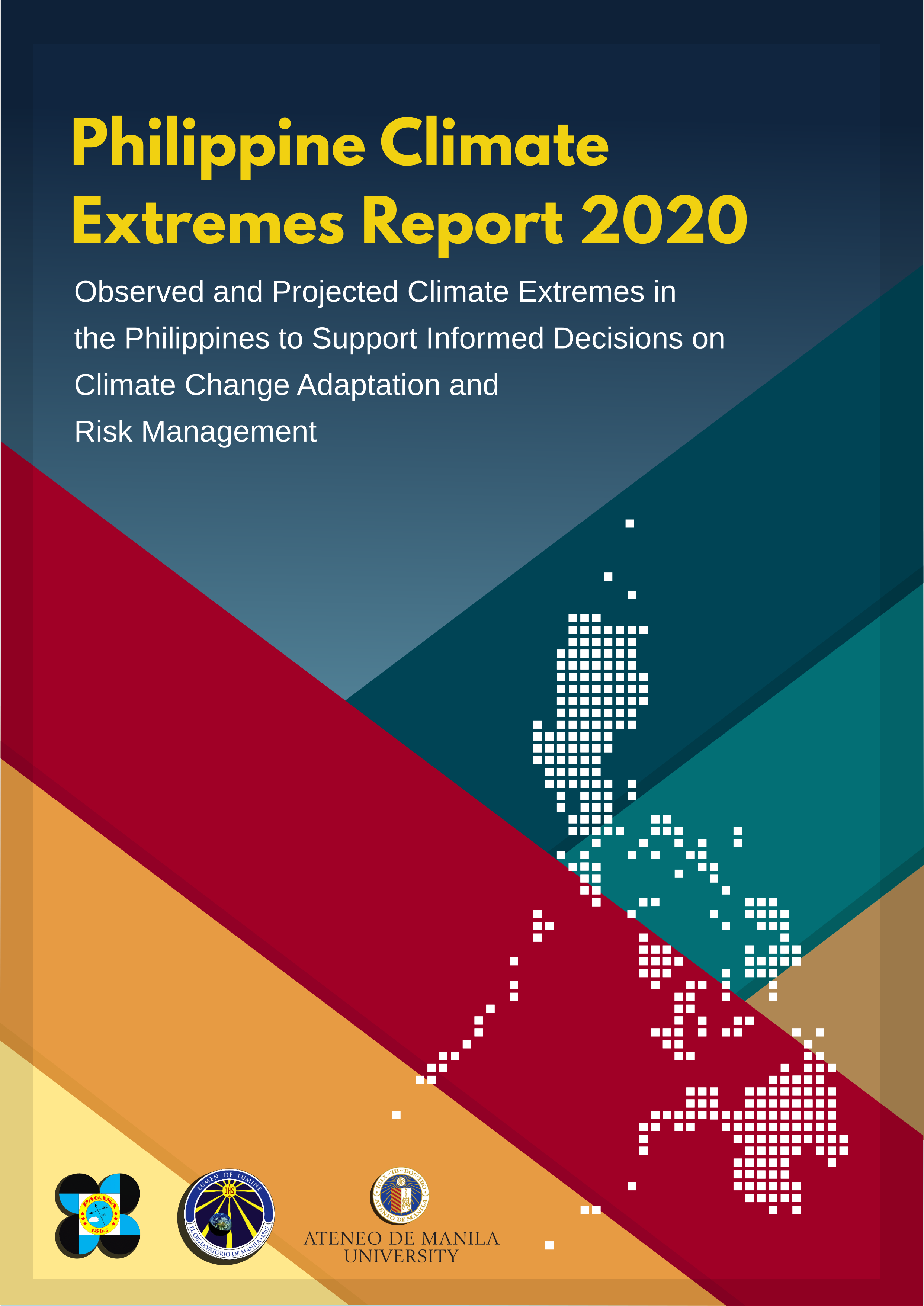
Sections
Download (4.8 MB)
Download (12.7 MB)
Download (7.6 MB)
Download (3.3 MB)
Annexes
Download (4.8 MB)
Download (12.7 MB)
AnnexesA: Provincial-scale Observed and Projected Annual Extremes
Download (1.6 MB)
Cordillera Administrative Region (CAR)
Download (5.8 MB)
Region I (Ilocos Region)
Download (4.1 MB)
Region II (Cagayan Valley)
Download (4.1 MB)
Region III (Central Luzon)
Download (6.6 MB)
Region IV-A (Calabarzon)
Download (4.9 MB)
Download (5.0 MB)
Region V (Bicol Region)
Download (5.8 MB)
Region VI (Western Visayas)
Download (4.9 MB)
Region VII (Central Visayas)
Download (3.3 MB)
Region VIII (Eastern Visayas)
Download (5.0 MB)
Region IX (Zamboanga Peninsula)
Download (3.3 MB)
Download (4.1 MB)
Region XI (Davao Region)
Download (4.1 MB)
Region XII (SOCCSKSARGEN)
Download (4.1 MB)
Region XIII (CARAGA)
Download (4.1 MB)
Bangsamoro Autonomus Region in Muslim Mindanao (BARMM)
Download (2.5 MB)
Climate change is happening now. Evidences being seen support the fact that the change cannot simply be explained by natural variation. The most recent scientific assessments have confirmed that this warming of the climate system since the mid-20th century is most likely to be due to human activities; and thus, is due to the observed increase in greenhouse gas concentrations from human activities, such as the burning of fossil fuels and land use change. Current warming has increasingly posed quite considerable challenges to man and the environment, and will continue to do so in the future. Presently, some autonomous adaptation is taking place, but we need to consider a more pro-active adaptation planning in order to ensure sustainable development.
What does it take to ensure that adaptation planning has a scientific basis? Firstly, we need to be able to investigate the potential consequences of anthropogenic or human induced climate change and to do this, a plausible future climate based on a reliable and accurate baseline (or present) climate must be constructed. This is what climate scientists call a climate change scenario. It is a projection of the response of the climate system to future emissions or concentrations of greenhouse gases and aerosols, and is simulated using climate models. Essentially, it describes possible future changes in climate variables (such as temperatures, rainfall, storminess, winds, etc.) based on baseline climatic conditions.
The climate change scenarios outputs (projections) are an important step forward in improving our understanding of our complex climate, particularly in the future. These show how our local climate could change dramatically should the global community fail to act towards effectively reducing greenhouse gas emissions.
As has been previously stated, climate change scenarios are developed using climate models (UNFCCC). These models use mathematical representations of the climate system, simulating the physical and dynamical processes that determine global/regional climate. They range from simple, one-dimensional models to more complex ones such as global climate models (known as GCMs), which model the atmosphere and oceans, and their interactions with land surfaces. They also model change on a regional scale (referred to as regional climate models), typically estimating change in areas in grid boxes that are approximately several hundred kilometers wide. It should be noted that GCMs/RCMs provide only an average change in climate for each grid box, although realistically climates can vary considerably within each grid. Climate models used to develop climate change scenarios are run using different forcings such as the changing greenhouse gas concentrations. These emission scenarios known as the SRES (Special Report on Emission Scenarios) developed by the Intergovernmental Panel on Climate Change (IPCC) to give the range of plausible future climate. These emission scenarios cover a range of demographic, societal, economic and technological storylines. They are also sometimes referred to as emission pathways. Table 1 presents the four different storylines (A1, A2, B1 and B2) as defined in the IPCC SRES.
Climate change is driven by factors such as changes in the atmospheric concentration of greenhouse gases and aerosols, land cover and radiation, and their combinations, which then result in what is called radiative forcing (positive or warming and negative or cooling effect). We do not know how these different drivers will specifically affect the future climate, but the model simulation will provide estimates of its plausible ranges.
A number of climate models have been used in developing climate scenarios. The capacity to do climate modeling usually resides in advanced meteorological agencies and in international research laboratories for climate modeling such as the Hadley Centre for Climate Prediction and Research of the UK Met Office (in the United kingdom), the National Center for Atmospheric Research and the Geophysical Fluid Dynamics Laboratory (in the United States), the Max Planck Institute for Meteorology (in Germany), the Canadian Centre for Climate Modeling and Analysis (in Canada), the Commonwealth Scientific and Industrial Research Organization (in Australia), the Meteorological Research Institute of the Japan Meteorological Agency (in Japan), and numerous others. These centers have been developing their climate models and continuously generate new versions of these models in order address the limitations and uncertainties inherent in models.

For the climate change scenarios in the Philippines presented in this Report, the PRECIS (Providing Regional Climates for Impact Studies) model was used. It is a PC-based regional climate model developed at the UK Met Office Hadley Centre for Climate Prediction and Research to facilitate impact, vulnerability and adaptation assessments in developing countries where capacities to do modeling are limited. Two time slices centered on 2020 (2006-2035) and 2050 (2036-2065) were used in the climate simulations using three emission scenarios; namely, the A2 (high-range emission scenario), the A1B (medium- range emission scenario) and the B2 (low-range emission scenario).
The high-range emission scenario connotes that society is based on self-reliance, with continuously growing population, a regionally-oriented economic development but with fragmented per capita economic growth and technological change. On the other hand, the mid-range emission scenario indicates a future world of very rapid economic growth, with the global population peaking in mid-century and declining thereafter and there is rapid introduction of new and more efficient technologies with energy generation balanced across all sources. The low-range emission scenario, in contrast, indicates a world with local solutions to economic, social, and environmental sustainability, with continuously increasing global population, but at a rate lower than of the high-range, intermediate levels of economic development, less rapid and more diverse technological change but oriented towards environment protection and social equity.
To start the climate simulations or model runs, outputs (climate information) from the relatively coarse resolution GCMs are used to provide high resolution (using finer grid boxes, normally 10km-100km) climate details, through the use of downscaling techniques. Downscaling is a method that derives local to regional scale (10km-100km x 10km-100km grids) information from larger-scale models (150km-300km x 150km-300km grids) as shown in Fig.1. The smaller the grid, the finer is the resolution giving more detailed climate information.
The climate simulations presented in this report used boundary data that were from the ECHAM4 and HadCM3Q0 (the regional climate models used in the PRECIS model software).

To run regional climate models, boundary conditions are needed in order to produce local climate scenarios. These boundary conditions are outputs of the GCMs. For the PRECIS model, the following boundary data and control runs were used:
For the high-range scenario, the GCM boundary data used was from ECHAM4. This is the 4th generation coupled ocean-atmosphere general circulation model, which uses a comprehensive parameterization package developed at the Max Planck Institute for Meteorology in Hamburg, Germany. Downscaling was to a grid resolution of 25km x 25km; thus, allowing more detailed regional information of the projected climate. Simulated baseline climate used for evaluation of the models capacity of reproducing present climate was the 1971-2000 model run. Its outputs were compared with the 1971-2000 observed values.
For the mid-range scenario, the GCM boundary data was from the HadCM3Q0 version 3 of the coupled model developed at the Hadley Centre. Downscaling was also to a grid resolution of 25km x 25km and the same validation process was undertaken.
For running the low-range scenario, the same ECHAM4 model was used. However, the validation process was only for the period of 1989 to 2000 because the available GCM boundary data in the model was limited to this period.
The simulations for all 3 scenarios were for three periods; 1971 to 2000, 2020 and 2050. The period 1971 to 2000 simulation is referred to as the baseline climate, outputs of which are used to evaluate the models capacity of reproducing present climate (in other words, the control run). By comparing the outputs (i.e., temperature and rainfall) with the observed values for the 1971 to 2000 period, the models ability to realistically represent the regional climatological features within the country is verified. The differences between the outputs and the observed values are called the biases of the model. The 2020 and 2050 outputs are then mathematically corrected, based on the comparison of the models performance.
The main outputs of the simulations for the three SRES scenarios (high-range, mid-range and low-range) are the following:
- projected changes in seasonal and annual mean temperature
- projected changes in minimum and maximum temperatures
- projected changes in seasonal rainfall and
- projected frequency of extreme events
The seasonal variations are as follows:
- the DJF (December, January, February or northeast monsoon locally known as amihan) season
- the MAM (March, April, May or summer) season
- the JJA (June, July, August or southwest monsoon season, or habagat) season and
- the SON (September, October, November or transition from southwest to northeast monsoon) season
On the other hand, extreme events are defined as follows:
- extreme temperature (assessed as number of days with maximum temperature greater than 35°C, following the threshold values used in other countries in the Asia Pacific region)
- dry days (assessed as number of dry days or day with rainfall equal or less than 2.5mm/day, following the World Meteorological Organization standard definition of dry days used in a number of countries) and
- extreme rainfall (assessed as number of days with daily rainfall greater than 300mm, which for wet tropical areas, like the Philippines, is considerably intense that could trigger disastrous events).
Modeling of our future climate always entails uncertainties. These are inherent in each step in the simulations/modeling done because of a number of reasons. Firstly, emissions scenarios are uncertain. Predicting emissions is largely dependent on how we can predict human behavior, such as changes in population, economic growth, technology, energy availability and national and international policies (which include predicting results of the international negotiations on reducing greenhouse gas emissions). Secondly, current understanding of the carbon cycle and of sources and sinks of non-carbon greenhouse gases are still incomplete. Thirdly, consideration of very complex feedback processes in the climate system in the climate models used can also contribute to the uncertainties in the outputs generated as these could not be adequately represented in the models.
But while it is difficult to predict global greenhouse gas emission rates far into the future, it is stressed that projections for up to 2050 show little variation between different emission scenarios, as these near-term changes in climate are strongly affected by greenhouse gases that have already been emitted and will stay in the atmosphere for the next 50 years. Hence, for projections for the near-term until 2065, outputs of the mid-range emission scenario are presented in detail in this Report.
Ideally, numerous climate models and a number of the emission scenarios provided in the SRES should be used in developing the climate change scenarios in order to account for the limitations in each of the models used, and the numerous ways global greenhouse gas emissions would go. The different model outputs should then be analyzed to calculate the median of the future climate projections in the selected time slices. By running more climate models for each emission scenarios, the higher is the statistical confidence in the resulting projections as these constitute the ensemble representing the median values of the model outputs.
The climate projections for the three emission scenarios were obtained using the PRECIS model only due to several constraints and limitations. These constraints and limitations are:
Access to climate models: at the start, PAGASA had not accessed climate models due to computing and technical capacity requirements needed to run them;
Time constraints: the use of currently available computers required substantial computing time to run the models (measured in weeks and months). This had been partly addressed under the capacity upgrading initiatives being implemented by the MDGF Joint Programme which include procurement of more powerful computers and acquiring new downscaling techniques. Improved equipment and new techniques have reduced the computing time requirements to run the models. However, additional time is still needed to run the models using newly acquired downscaling techniques; and
The PAGASA strives to improve confidence in the climate projections and is continuously exerting efforts to upgrade its technical capacities and capabilities. Models are run as soon as these are acquired with the end-goal of producing an ensemble of the projections. Updates on the projections, including comparisons with the current results, will be provided as soon as these are available.
The IPCC stresses that there is a large degree of uncertainty in predicting what the future world will be despite taking into account all reasonable future developments. Nevertheless, there is high confidence in the occurrence of global warming due to emissions of greenhouse gases caused by humans, as affirmed in the IPCC Fourth Assessment Report (AR4). Global climate simulations done to project climate scenarios until the end of the 21st century indicate that, although there are vast differences between the various scenarios, the values of temperature increase begin to diverge only after the middle of this century (shown in Fig.3). The long lifetimes of the greenhouse gases (in particular, that of carbon dioxide) already in the atmosphere is the reason for this behavior of this climate response to largely varying emission scenarios.

Model outputs that represent the plausible local climate scenarios in this Report are indicative to the extent that they reflect the large-scale changes (in the regional climate model used) modified by the projected local conditions in the country.
It also should be stressed further that confidence in the climate change information depends on the variable being considered (e.g., temperature increase, rainfall change, extreme event indices, etc.). In all the model runs regardless of emission scenarios used, there is greater confidence in the projections of mean temperature than that of the others. On the other hand, projections of rainfall and extreme events entail consideration of convective processes which are inherently complex, and thus, limiting the degree of confidence in the outputs.
Climate scenarios are commonly required in climate change impact, vulnerability and adaptation assessments to provide alternative views of future conditions considered likely to affect society, systems and sectors, including a quantification of climate risks, challenges and opportunities. Climate scenario outputs could be used in any of the following:
- to illustrate projected climate change in a given administrative region/province
- to provide data for impact/adaptation assessment studies
- to communicate potential consequences of climate change (e.g., specifying a future changed climate to estimate potential shifts in say, vegetation, species threatened or at risk of extinction, etc.) and
- for strategic planning (e.g., quantifying projected sea level rise and other climate changes for the design of coastal infrastructure/defenses such as sea walls, etc.)
The world has increasingly been concerned with the changes in our climate due largely to adverse impacts being seen not just globally, but also in regional, national and even, local scales. In 1988, the United Nations established the IPCC to evaluate the risks of climate change and provide objective information to governments and various communities such as the academe, research organizations, private sector, etc. The IPCC has successively done and published its scientific assessment reports on climate change, the first of which was released in 1990. These reports constitute consensus documents produced by numerous lead authors, contributing authors and review experts representing Country Parties of the UNFCCC, including invited eminent scientists in the field from all over the globe.
In 2007, the IPCC made its strongest statement yet on climate change in its Fourth Assessment Report (AR4), when it concluded that the warming of the climate system is unequivocal, and that most of the warming during the last 50 years or so (e.g., since the mid-20th century) is due to the observed increase in greenhouse gas concentrations from human activities. It is also very likely that changes in the global climate system will continue into the future, and that these will be larger than those seen in our recent past (IPCC, 2007a).
Fig.4 shows the 0.74 C increase in global mean temperature during the last 150 years compared with the 1961-1990 global average. It is the steep increase in temperature since the mid-20th century that is causing worldwide concern, particularly in terms of increasing vulnerability of poor developing countries, like the Philippines, to adverse impacts of even incremental changes in temperatures.


The IPCC AR4 further states that the substantial body of evidence that support this most recent warming includes rising surface temperature, sea level rise and decrease in snow cover in the Northern Hemisphere (shown in Fig.5).
Additionally, there have been changes in extreme events globally and these include;
- widespread changes in extreme temperatures observed;
- cold days, cold nights and frost becoming less frequent;
- hot days, hot nights and heat waves becoming more frequent; and
- observational evidence for an increase of intense tropical cyclone activity in the North Atlantic since about 1970, correlated with increases of tropical sea surface temperatures (SSTs).
However, there are differences between and within regions. For instance, in the Southeast Asia region which includes Indonesia, Malaysia, the Philippines, Thailand, and Vietnam, among others, temperature increases have been observed; although magnitude varies from one country to another. Changes in rainfall patterns, characteristically defined by changes in monsoon performance, have also been noted. Analysis of trends of extreme daily events (temperatures and rainfall) in the Asia Pacific region (including Australia and New Zealand, and parts of China and Japan) also indicate spatial coherence in the increase of hot days, warm nights and heat waves, and the decrease of cold days, cold nights and frost; although, there is no definite direction of rainfall change across the entire region (Manton et. al., 2001).
The Philippines, like most parts of the globe, has also exhibited increasing temperatures as shown in Fig.6 below. The graph of observed mean temperature anomalies (or departures from the 1971-2000 normal values) during the period 1951 to 2010 indicate an increase of 0.648 C or an average of 0.0108 C per year-increase.

The increase in maximum (or daytime) temperatures and minimum (or night time) temperatures are shown in Fig.7 and Fig.8. During the last 60 years, maximum and minimum temperatures are seen to have increased by 0.36 ºC and 1.0°C, respectively.


Analysis of trends of tropical cyclone occurrence or passage within the so-called Philippine Area of Responsibility (PAR) show that an average of 20 tropical cyclones form and/or cross the PAR per year. The trend shows a high variability over the decades but there is no indication of increase in the frequency. However, there is a very slight increase in the number of tropical cyclones with maximum sustained winds of greater than 150kph and above (typhoon category) being exhibited during El NiÑo event (See Fig.10).


Moreover, the analysis on tropical cyclone passage over the three main islands (Luzon, Visayas and Mindanao), the 30-year running means show that there has been a slight increase in the Visayas during the 1971 to 2000 as compared with the 1951 to 1980 and 1960-1990 periods (See Fig.11).

To detect trends in extreme daily events, indices had been developed and used. Analysis of extreme daily maximum and minimum temperatures (hot-days index and cold-nights index, respectively) show there are statistically significant increasing number of hot days but decreasing number of cool nights (as shown in Fig.12 and Fig.13).

However, the trends of increases or decreases in extreme daily rainfall are not statistically significant; although, there have been changes in extreme rain events in certain areas in the Philippines. For instance, intensity of extreme daily rainfall is already being experienced in most parts of the country, but not statistically significant (see in Fig.14). Likewise, the frequency has exhibited an increasing trend, also, not statistically significant (as shown in Fig.15).

The rates of increases or decreases in the trends are point values (i.e., specific values in the synoptic weather stations only) and are available at PAGASA, if needed.
Projections on seasonal temperature increase and rainfall change, and total frequency of extreme events nationally and in the provinces using the mid-range scenario outputs are presented in this chapter. A comparison of these values with the high- and low- range scenarios in 2020 and 2050 is provided in the technical annexes.
It is to be noted that all the projected changes are relative to the baseline (1971-2000) climate. For example, a projected 1.0 C-increase in 2020 in a province means that 1.0 C is added to the baseline mean temperature value of the province as indicated in the table to arrive at the value of projected mean temperature. Therefore, if the baseline mean temperature is 27.8 C, then the projected mean temperature in the future is (27.8 C + 1.0 C) or 28.8 C.
In a similar manner, for say, a +25%-rainfall change in a province, it means that 25% of the seasonal mean rainfall value in the said province (from table of baseline climate) is added to the mean value. Thus, if the baseline seasonal rainfall is 900mm, then projected rainfall in the future is 900mm + 225mm or 1125mm.
This means that we are already experiencing some of the climate change shown in the findings under the mid-range scenario, as we are now into the second decade of the century. Classification of climate used the Corona's four climate types (Types I to IV), based on monthly rainfall received during the year. A province is considered to have Type I climate if there is a distinct dry and a wet season; wet from June to November and dry, the rest of the year. Type II climate is when there is no dry period at all throughout the year, with a pronounced wet season from November to February. On the other hand, Type III climate is when there is a short dry season, usually from February to April, and Type IV climate is when the rainfall is almost evenly distributed during the whole year. The climate classification in the Philippines is shown in Fig.16.
All areas of the Philippines will get warmer, more so in the relatively warmer summer months. Mean temperatures in all areas in the Philippines are expected to rise by 0.9 C to 1.1 C in 2020 and by 1.8 C to 2.2 C in 2050. Likewise, all seasonal mean temperatures will also have increases in these time slices; and these increases during the four seasons are quite consistent in all parts of the country. Largest temperature increase is projected during the summer (MAM) season.

Generally, there is reduction in rainfall in most parts of the country during the summer (MAM) season. However, rainfall increase is likely during the southwest monsoon (JJA) season until the transition (SON) season in most areas of Luzon and Visayas, and also, during the northeast monsoon (DJF) season, particularly, in provinces/areas characterized as Type II climate in 2020 and 2050. There is however, generally decreasing trend in rainfall in Mindanao, especially by 2050.
There are varied trends in the magnitude and direction of the rainfall changes, both in 2020 and 2050. What the projections clearly indicate are the likely increase in the performance of the southwest and the northeast monsoons in the provinces exposed to these climate controls when they prevail over the country. Moreover, the usually wet seasons become wetter with the usually dry seasons becoming also drier; and these could lead to more occurrences of floods and dry spells/droughts, respectively.

Hot temperatures will continue to become more frequent in the future. Fig.19 shows that the number of days with maximum temperature exceeding 35 C (following value used by other countries in the Asia Pacific region in extreme events analysis) is increasing in 2020 and 2050.

Heavy daily rainfall will continue to become more frequent, extreme rainfall is projected to increase in Luzon and Visayas only, but number of dry days is expected to increase in all parts of the country in 2020 and 2050. Figures 20 and 21 show the projected increase in number of dry days (with dry day defined as that with rainfall less than 2.5mm) and the increase in number of days with extreme rainfall (defined as daily rainfall exceeding 300 mm) compared with the observed (baseline) values, respectively.
Heavy daily rainfall will continue to become more frequent, extreme rainfall is projected to increase in Luzon and Visayas only, but number of dry days is expected to increase in all parts of the country in 2020 and 2050. Figures 20 and 21 show the projected increase in number of dry days (with dry day defined as that with rainfall less than 2.5mm) and the increase in number of days with extreme rainfall (defined as daily rainfall exceeding 300 mm) compared with the observed (baseline) values, respectively.
Climate change is one of the most fundamental challenges ever to confront humanity. Its adverse impacts are already being seen and may intensify exponentially over time if nothing is done to reduce further emissions of greenhouse gases. Decisively dealing NOW with climate change is key to ensuring sustainable development, poverty eradication and safeguarding economic growth. Scientific assessments indicate that the cost of inaction now will be more costly in the future. Thus, economic development needs to be shifted to a low-carbon emission path.
In 1992, the United Nations Framework Convention on Climate Change (UNFCCC) was adopted as the basis for a global response to the problem. The Philippines signed the UNFCCC on 12 June 1992 and ratified the international treaty on 2 August 1994. Presently, the Convention enjoys near-universal membership, with 194 Country Parties.
Recognizing that the climate system is a shared resource which is greatly affected by anthropogenic emissions of greenhouse gases, the UNFCCC has set out an overall framework for intergovernmental efforts to consider what can be done to reduce global warming and to cope with whatever temperature increases are inevitable. Its ultimate objective is to stabilize greenhouse gas concentrations in the atmosphere at a level that will prevent dangerous human interference with the climate system.
Countries are actively discussing and negotiating ways to deal with the climate change problem within the UNFCCC using two central approaches. The first task is to address the root cause by reducing greenhouse gas emissions from human activity. The means to achieve this are very contentious, as it will require radical changes in the way many societies are organized, especially in respect to fossil fuel use, industry operations, land use, and development. Within the climate change arena, the reduction of greenhouse gas emissions is called mitigation.
The second task in responding to climate change is to manage its impacts. Future impacts on the environment and society are now inevitable, owing to the amount of greenhouse gases already in the atmosphere from past decades of industrial and other human activities, and to the added amounts from continued emissions over the next few decades until such time as mitigation policies and actions become effective. We are therefore committed to changes in the climate. Taking steps to cope with the changed climate conditions both in terms of reducing adverse impacts and taking advantage of potential benefits is called adaptation.
Responses of the local climate to the mid-range compared to the high- and low-range scenarios are as shown in Fig. 22 below. Although there are vast differences in the projections, the so-called temperature anomalies or difference in surface temperature increase begin to diverge only in the middle of the 21st century. As has already been stated, the climate in the next 30 to 40 years is greatly influenced by past greenhouse gas emissions. The long lifetimes of the greenhouse gases already in the atmosphere, with the exception of methane (with a lifetime of only 13 years), will mean that it will take at least 30 to 40 years for the atmosphere to stabilize even if mitigation measures are put in place, not withstanding that in the near future, there could be some off-setting between sulfate aerosols (cooling effect) and the greenhouse gas concentrations (warming effect).
A warmer world is certain to impact on systems and sectors; although, magnitude of impacts will depend on factors such as sensitivity, exposure and adaptive capacity to climate risks. In most cases, likely impacts will be adverse. However, there could be instances when likely impacts present opportunities for potential benefits as in the case of the so-called carbon fertilization effect in which increased carbon dioxide could lead to increased yield provided temperatures do not exceed threshold values for a given crop/cultivar.
In areas/regions where rainfall is projected to decrease, there will be water stress (both in quantity and quality), which in turn, will most likely cascade into more adverse impacts, particularly on forestry, agriculture and livelihood, health, and human settlement. Large decreases in rainfall and longer drier periods will affect the amount of water in watersheds and dams which provide irrigation services to farmers, especially those in rain fed areas, thereby, limiting agricultural production. Likewise, energy production from dams could also be rendered insufficient in those areas where rainfall is projected to decrease, and thus, could largely affect the energy sufficiency program of the country. Design of infrastructure, particularly of dams, will need to be re-visited to ensure that these will not be severely affected by the projected longer drier periods.

In areas where rainfall could be intense during wet periods, flooding events would follow and may pose danger to human settlements and infrastructure, in terms of landslides and mudslides, most especially, in geologically weak areas. Additionally, these flooding events could impact severely on public infrastructure, such as roads and bridges, including classrooms, evacuation centers, and hospitals.
Adaptive capacity is enhanced when impact and vulnerability assessments are used as the basis of strategic and long-term planning for adaptation. Assessments would indicate areas where critical water shortages can be expected leading to possible reduction of water available for domestic consumption, less irrigation service delivery, and possibly, decreased energy generation in dams. Note that the adverse impacts would cascade, so that long-term pro-active planning for these possible impacts is imperative in order to be able to respond effectively, and avoid maladaptations. A number of adaptation strategies should be considered. Among the wide array of cost effective options are rational water management, planning to avoid mismatch between water supply and demand through policies, upgrading/rehabilitation of dams where these are cost-effective, changes in cropping patterns in agricultural areas, establishing rain water collection facilities, where possible, and early warning systems.
Changes in rainfall regimes and patterns resulting to increase/decrease in water use and temperature increases could lead to a change in the forests ecosystem, particularly in areas where the rains are severely limited, and can no longer provide favorable conditions for certain highly sensitive species. Some of our forests could face die-backs. Additionally, drier periods and warmer temperatures, especially during the warm phase of El Nino events, could cause forest fires. A very likely threat to communities that largely depend on the ecological services provided by forests is that they may face the need to alter their traditions and livelihoods. This change in practices and behavior can lead to further degradation of the environment as they resort to more extensive agricultural production in already degraded areas.

Adverse impacts on forestry areas and resources could be expected to multiply in a future warmer world. The value of impact and vulnerability assessments could not be underscored. These assessments would help decision makers and stakeholders identify the best option to address the different impacts on forest areas, watersheds and agroforestry. Indigenous communities have to plan for climate-resilient alternative livelihoods. Thus, it is highly important to plan for rational forest management, particularly, in protected areas and in ancestral domains. One of the more important issues to consider is how to safeguard livelihoods in affected communities so as not to further exacerbate land degradation. Early warning systems in this sector will play a very important role in forest protection through avoidance and control/containment of forest fires.
Agriculture in the country could be severely affected by temperature changes coupled with changes in rain regimes and patterns. Crops have been shown to suffer decreases in yields whenever temperatures have exceeded threshold values and possibly result to spikelet sterility, as in the case of rice. The reduction in crop yield would remain unmitigated or even aggravated if management technologies are not put in place. Additionally, in areas where rain patterns change or when extreme events such as floods or droughts happen more often, grain and other agricultural produce could suffer shortfalls in the absence of effective and timely interventions. Tropical cyclones, particularly if there will be an increase in numbers and/or strength will continue to exert pressure on agricultural production.
Moreover, temperature increases coupled with rainfall changes could affect the incidence/outbreaks of pests and diseases, both in plants and animals. The pathways through which diseases and pests could be triggered and rendered most favorable to spread are still largely unknown. It is therefore important that research focus on these issues.

In the fisheries sub-sector, migration of fish to cooler and deeper waters would force the fisher folks to travel further from the coasts in order to increase their catch. Seaweed production, already being practiced as an adaptation to climate change in a number of poor and depressed coastal communities could also be impacted adversely.
Decreased yields and inadequate job opportunities in the agricultural sector could lead to migration and shifts in population, resulting to more pressure in already depressed urban areas, particularly in mega cities. Food security will largely be affected, especially if timely, effective and efficient interventions are not put in place. Insufficient food supply could further lead to more malnutrition, higher poverty levels, and possibly, heightened social unrest and conflict in certain areas in the country, and even among the indigenous tribes.
A careful assessment of primary and secondary impacts in this sector, particularly, in production systems and livelihoods will go a long way in avoiding food security and livelihood issues. Proactive planning (short- and long-term adaptation measures) will help in attaining poverty eradication, sufficient nutrition and secure livelihoods goals. There is a wide cross-section of adaptation strategies that could be put in place, such as horizontal and vertical diversification of crops, farmer field schools which incorporate use of weather/climate information in agricultural operations, including policy environment for subsidies and climate-friendly agricultural technologies, weather-based insurance, and others. To date, there has not been much R&D that has been done on inland and marine fisheries technologies, a research agenda on resilient marine sector could form part of long-term planning for this subsector.
The countrys coastal resources are highly vulnerable due to its extensive coastlines. Sea level rise is highly likely in a changing climate, and low-lying islands will face permanent inundation in the future. The combined effects of continued temperature increases, changes in rainfall and accelerated sea level rise, and tropical cyclone occurrences including the associated storm surges would expose coastal communities to higher levels of threat to life and property. The livelihood of these communities would also be threatened in terms of further stress to their fishing opportunities, loss of productive agricultural lands and saltwater intrusion, among others.
Impact and vulnerability assessment as well as adaptation planning for these coastal areas are of high priority. Adaptation measures range from physical structures such as sea walls where they still are cost-effective, to development/revision of land use plans using risk maps as the basis, to early warning systems for severe weather, including advisories on storm surge probabilities, as well as planning for and developing resilient livelihoods where traditional fishing/ agriculture are no longer viable.

Human health is one of the most vital sectors which will be severely affected by climate change. Incremental increases in temperatures and rain regimes could trigger a number of adverse impacts; in particular, the outbreak and spread of water-based and vector-borne diseases leading to higher morbidity and mortality; increased incidence of pulmonary illnesses among young children and cardiovascular diseases among the elderly. In addition, there could also be increased health risk from poor air quality especially in urbanized areas.
Surveillance systems and infrastructure for monitoring and prevention of epidemics could also be under severe stress when there is a confluence of circumstances. Hospitals and clinics, and evacuation centers and resettlement areas could also be severely affected under increased frequency and intensity of severe weather events.

Moreover, malnutrition is expected to become more severe with more frequent occurrences of extreme events that disrupt food supply and provision of health services. The services of the Department of Health will be severely tested unless early and periodic assessments of plausible impacts of climate change are undertaken.
Scientific assessments have indicated that the Earth is now committed to continued and faster warming unless drastic global mitigation action is put in place the soonest. The likely impacts of climate change are numerous and most could seriously hinder the realization of targets set under the Millennium Development Goals; and thus, sustainable development. Under the UNFCCC, Country Parties have common but differentiated responsibilities. All Country Parties share the common responsibility of protecting the climate system but must shoulder different responsibilities. This means that the developed countries including those whose economies are in transition (or the so-called Annex 1 Parties) have an obligation to reduce their greenhouse gas emissions based on their emissions at 1990 levels and provide assistance to developing countries (or the so-called non-Annex 1 Parties) to adapt to impacts of climate change.

In addition, the commitment to mitigate or reduce anthropogenic greenhouse gas emissions by countries which share the responsibility of having historically caused this global problem, as agreed upon in the Kyoto Protocol, is dictated by the imperative to avoid what climate scientists refer to as the climate change tipping point. Tipping point is defined as the maximum temperature increase that could happen within the century, which could lead to sudden and dramatic changes to some of the major geophysical elements of the Earth. The effects of these changes could be varied from a dramatic rise in sea levels that could flood coastal regions to widespread crop failures. But, it still is possible to avoid them with cuts in anthropogenic greenhouse gases, both in the developed and developing countries, in particular, those which are now fast approaching the emission levels seen in rich countries.
In the Philippines, there are now a number of assisted climate change adaptation programmes and projects that are being implemented. Among these are the Millennium Development Goals Fund 1656: Strengthening the Philippines Institutional Capacity to Adapt to Climate Change funded by the Government of Spain, the Philippine Climate Change Adaptation Project (which aims to develop the resiliency and test adaptation strategies that will develop the resiliency of farms and natural resource management to the effects of climate change) funded by the Global Environmental Facility(GEF) through the World Bank, the Adaptation to Climate Change and Conservation of Biodiversity Project and the National Framework Strategy on Climate Change (envisioned to develop the adaptation capacity of communities), both funded by the GTZ, Germany.